Irrigation adaptations are leaving surface and groundwater cleaner. It’s good for the environment, downstream water users, crops, and producers’ bottom line
On the edge of Larry Lempka’s farmland, where it slopes down to the Little Thompson River in northern Colorado, you stand in a miniature tallgrass prairie. Orchardgrass, brome grass and fescue sway in the wind as if petted by an invisible hand, their broad blades reflecting the sunlight as they bend.
This mini-prairie only spans 100 to 300 feet from the water’s edge before it gives way to rows of forage sorghum or sunflowers, corn or potatoes, depending on Lempka’s rotation. But Lempka suspected that the grasses he planted protected the river from irrigation runoff generated by his fields and those of his neighbors, since his plot stands between theirs and the Little Thompson River. In 2018, he asked the College of Agricultural Sciences at Colorado State University to measure the grasses’ effect.
“I’m scientific,” explains Lempka, who’s worked these lands near Berthoud for 51 years. He knew the grasses would prevent erosion, but he wasn’t so sure about their effectiveness at removing silt and nutrients from the irrigation runoff that returned to the river. “How much difference is there? What’s our impact?” Lempka wondered.
Erik Wardle, manager of CSU’s Agricultural Water Quality Program, worked with Lempka to install water-monitoring units located at the crops’ border with the grasses near the river’s edge. The monitoring stations let researchers compare the water’s quality before it reaches the grass strip and again before it enters the Little Thompson. Results vary by season, but overall, Wardle summarizes, the mini-prairie “filter strip” proved to be extremely effective at removing sediment and achieved significant reductions to total phosphorus levels reaching the river. Too much phosphorus or other nutrients can cause algae blooms, reducing water-oxygen levels and harming fish and aquatic life. Such blooms can also produce toxins poisonous to animals and humans.
Some mysteries remain to be solved—for example, E. coli, a known impairment along this stretch of the Little Thompson, fluctuates according to factors that aren’t currently well understood—but the data suggests that scaling up the grass-strip design along more farmlands within the Little Thompson and South Platte watersheds could have significant benefits to water quality by limiting the quantities of silt and nutrients that dump into the rivers. That evidence could embolden entities such as the Natural Resources Conservation Service (NRCS), a federal office that funds environmental improvement projects on private land, to invest in grass filter strip installations beyond Lempka’s half-mile of river frontage. The potential excites Wardle. “What would the impact be if 10 farms, or 50 farms employed these filter strips along the river?”

Lempka Family Farm is nestled beside the Little Thompson River. To effectively intercept irrigation runoff and remove sediment and nutrients before they enter the river, Larry Lempka installed a vegetative filter strip abutting the river. Photo by Christi Bode Skeie courtesy of Colorado’s Water Quality Control Division Nonpoint Source Program
Across Colorado, surface waters and underground water sources contain various contaminants that can result in what’s known as water quality impairments, where those sources don’t meet water quality standards. Some impairments are driven by agricultural irrigation and runoff. Excess nitrogen and phosphorus from fertilizers, including compost and manure, have the potential to run into streams and leach into groundwater. The same is true of herbicides and pesticides. Other regions experience elevated levels of salt and selenium in their fields, rivers and streams. Often, geology is to blame: Many millions of years ago, inland seas covered much of what is now Colorado, depositing limestone, shale and sandstone. Today, as irrigation water and natural flows filter through different formations, including the Mancos shale that underlies much of Colorado, the water unlocks selenium and uranium. When that water is applied it can broadcast those elements across fields, harming soils and plants, and wash them back into streams and groundwater—even reaching drinking water sources.
In other cases, water quality begins to degrade with use in urban areas from sources including wastewater, stormwater and landscape runoff. By the time that water has been used, reused and flows downstream to agricultural operations, it may be carrying higher loads of nutrients and pollutants.
EPA studies conducted nationwide confirm that agricultural runoff is the leading source of pollutants in surveyed rivers and lakes. Those pollutants can pose unfortunate consequences for human health and economies. Removal and regulation of these nonpoint source pollutants aren’t great options: It’s whoppingly expensive to build wastewater treatment plants capable of removing salt, uranium and other contaminants from drinking water, and nearly impossible to measure and regulate agricultural runoff. Each farm applies different levels of fertilizers, pesticides and irrigation water at different times to different types of soil and various crops—all factors that affect the way pollutants leave a field. Plus runoff from fields occurs in various places, and groundwater leaching is traceable only through research—it would be a challenge to accurately measure the rate of pollutants leaving agricultural systems. High levels of salinity can inhibit crop production. And extended exposure to high concentrations of selenium, uranium and nitrate cause a variety of human health issues. While applied irrigation water isn’t solely to blame, it can increase the rate in which naturally occurring elements are released and contaminate water.
Studies conducted over the past three decades have shed light on these ecological processes and are helping managers target the irrigation adaptations likely to yield the greatest benefit to water quality. Agricultural producers are doing their part: They’re turning out in high numbers to attend courses and learn strategies for improving water quality, as well as efficiency—the two can be correlated. And many farmers are implementing best management practices (BMPs) that are already achieving quantifiable gains. One example: In June 2021, the Lower Gunnison River was removed from the state’s impaired waters list after measurable improvement in its levels of dissolved selenium, which can become toxic for natural ecosystems and aquatic and human life in high concentrations.
Some growers are motivated by a desire to stave off regulation, and many are chasing the cost savings that can result from efficient irrigation practices. For example, when fertilizers stay in the ground rather than getting flushed into waterways, farmers enjoy higher yields while spending less on those inputs. Colorado’s health department has also been supporting water quality improvement projects that are typically driven by agriculture. “It’s an interesting time right now, because ag really wants to be involved in the conversation about water quality,” says Wardle. “We’re seeing a really positive collaboration between ag, researchers and the regulatory community.”
Challenges with Applied Water
Colorado growers don’t get a lot of natural rainfall: Totals vary from 7 inches of annual precipitation in the San Luis Valley to 15 inches in Weld County to 22 inches in Steamboat Springs, and higher at higher elevations where few crops are grown. Thus supplemental irrigation is required for commercial production of all but the most drought-tolerant crops, such as beans and wheat, which can be dry-farmed. But dryland farming works best in areas that receive higher amounts of rainfall and produces lower yields that result in less income for farmers. Strategies for watering crops date back to the state’s pioneer era.
Settlers built ditches and canals that channel water from rivers to fields, using headgates or siphon tubes to send flows across open fields or into trenches in the soil between rows of crops. Such low-tech “flood irrigation” methods are affordable to build and operate, thus they still represent about 43% to 49% of Colorado’s irrigation designs, estimates Wardle. But flood irrigation also carries away topsoil, can move sediment into streams, and increases the likelihood that irrigation water will pick up naturally occurring elements such as uranium or selenium.
Over time, all methods of water application—including the pressurized sprinklers that have replaced flood irrigation on many farms—alter the way that minerals and nutrients pass through the soil and enter surface and groundwater. Applied water can load the ground with salts that may compromise productivity and water quality. Irrigation can also flush phosphorus, nitrogen and other fertilizers into streams and groundwater, leading to what’s known as “nutrient pollution,” which can stimulate algae growth and in some cases lead to harmful algae blooms which can be toxic to fish, animals and humans. And when applied water hits the selenium-rich layer of cretaceous shale that underlies much of Colorado, it absorbs that mineral and transports it into water bodies, where it kills or deforms the larvae of aquatic animals. Uranium is another naturally occurring element that weathers out of Colorado’s marine shales. Evapotranspiration associated with irrigation and shallow reservoirs increases the uranium concentration of river water, according to 2016 research presented at the Geological Society of America annual meeting in Denver. At the same time, nitrates in water, the result of nitrogen fertilizers, may trigger uranium to degrade at a higher rate, becoming more soluble so that it contaminates groundwater. High concentrations of uranium in drinking water can cause cancer and kidney failure.
“Any activities that apply water to the landscape can impair surface and ground water,” explains Dave Kanzer, director of science and interstate matters for the Colorado River District. Such activities include residential septic systems and ornamental ponds. But irrigation is the state’s largest water use and according to computer models created by the selenium management program in the Lower Gunnison River Basin, irrigation is primarily responsible for releasing compounds such as selenium and uranium from soil to water. Therefore, improving approaches to irrigation can help clean up Colorado’s water.
Better water quality also requires the long view, says Kanzer, noting that the Lower Gunnison Basin effort involved a dedicated, core group that met on a monthly basis for nearly 15 years. Though funding sources for irrigation-related water quality projects can be complicated to coordinate, dollars are relatively available from entities such as the U.S. Bureau of Reclamation and NRCS, which have dedicated significant totals to battling salinity, which inhibits agricultural production and raises costs for water treatment plants, and selenium. Together, these entities have been contributing about $15-20 million annually since 2015 to projects on the Lower Gunnison that reduce pollution from salts and selenium. The money converted miles of open ditches to enclosed pipes and replaced flood irrigation systems with sprinklers across approximately 76,000 acres. All together, the widespread transformation to enclosed ditches and sprinkler systems brought the Lower Gunnison back into acceptable levels of selenium.
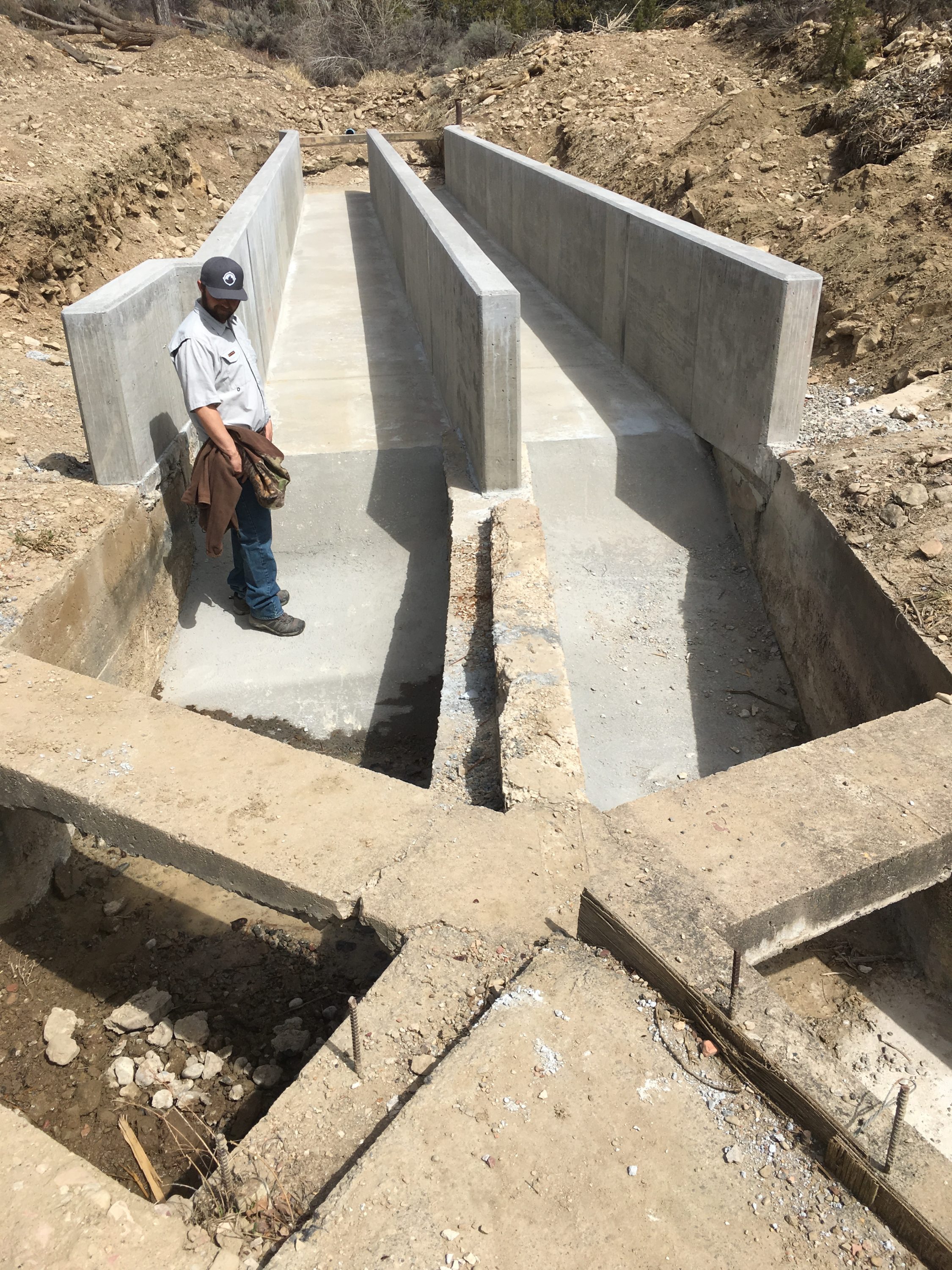
Silt Water Conservancy District superintendent Jason Spaulding inspects an upgraded water conveyance structure downstream from the Harvey Gap Reservoir, north of Rifle on Colorado’s Western Slope, in 2019. The structure was previously in disrepair and subject to leakage and water loss. Lined and piped conveyances, like this, significantly reduce water loss and seepage, and improve downstream water quality by minimizing the mobilization of naturally occurring selenium, salts and other contaminants from the subsurface to the river system.
Courtesy Dave “DK” Kanzer | Colorado River District
Most importantly, says Kanzer, “You’ve got to be committed to the science.” His project conducted an extensive GIS analysis of irrigation networks and compared that map against ones charting geologies with the highest potential for selenium mobilization. This overlay, along with chemical transport modeling, helped the selenium management team decide which irrigation upgrades—such as piping open ditches and converting from flood to sprinkler irrigation—would deliver the greatest benefit to water quality. Says Kanzer, “The science led us.”
Combatting Nutrient Pollution Through Ditch Seepage
Dr. Timothy Gates, Colorado State University Professor of Civil and Environmental Engineering, has been studying irrigation’s impact on water quality for more than 30 years, which is “longer than I’d like to admit,” he chuckles. Like Kanzer, he also views water quality improvement as a long game. “You can’t just go out there and effect change in a week or two,” he explains. Using models to understand how excess applied irrigation water migrates through the ecosystem and transports dissolved minerals, nutrients and other solutes, he’s seen that the water can take a few months to return to surface and groundwater resources—or even decades. Because of that lag, “It may take many years before you can see marked changes in water quality,” says Gates.
Much of his research was conducted along the Arkansas River, particularly the lower part of the river basin from Pueblo, Colo., to the border with Kansas. Originally, he studied the problem of salinity in those irrigated lands, but over the years, Gates and a team of dozens of CSU faculty, staff and students expanded the focus to address additional irrigation-induced water quality problems, such as waterborne selenium, uranium, nitrate and phosphate. Computational models developed by the CSU team allowed them to play imaginary “what if” games with various irrigation scenarios. Modeling generally confirmed the theory that too much applied water—more than is actually used by the crop—flushes fertilizer beyond the reach of plants’ roots, which wastes farmers’ money and loads groundwater with nutrients. Models also illustrated how this water containing nutrients liberates salts, selenium and uranium from Colorado’s shale layers and transports it into waterways.
But modeling also revealed a surprise: The greatest impairment to water quality in the aquifer and streams caused by agricultural irrigation came from seepage along the canals that divert water from rivers to fields. In the Arkansas Basin, and in many locations around the world, earthen canals transport water over vast tracts of lands that can span hundreds of thousands of acres. On average, says Gates, 25% to 30% of the water that’s diverted at the headgate never reaches the intended field to be used for irrigation because it’s lost to leakage along the way. Computational models projected significant reductions to pollutants in groundwater and rivers should that seepage be reduced, possibly by lining canals with concrete. Lining projects, however, cost many millions of dollars to improve just a few miles of canal.
Starting in 2006, Gates participated in a collaboration involving Reclamation and the Desert Research Institute that evaluated the possibility of treating canals using a polymer that binds with sediments and seals the channel. Over the past 15 years, lab studies and in-field pilot projects on canals along the Arkansas River and other locations have concluded that these polymers reduce seepage by as much as 80% and cost just 5% of the typical expense for concrete or plastic linings. That equates to significant gains in water quality: Modeling studies indicate that in the Arkansas River Basin sealing canals with polymers would reduce selenium and nitrate concentrations in the groundwater aquifer by about 18% and 11%, respectively, and would reduce selenium concentrations in the Arkansas River by up to 20%.
One tradeoff is the need to reapply polymer sealants every growing season, at minimum, whereas traditional concrete-lined or piped ditches should last at least 10 years, according to the NRCS, often much longer. Another downside is the polymer’s petrochemical makeup (one petrochemical polymer breaks down into compounds that can become carcinogenic in high concentrations). However, Gates, along with CSU Professor Joseph Scalia and PhD student Rehman Lund, is already testing a bio-based alternative made of xanthan gum, a common food additive. In 2021, a pilot project on the Larimer and Weld Canal near Fort Collins demonstrated that the biodegradable xanthan gum polymer was very effective at reducing seepage. The CSU team will repeat the test project on the same canal this year.

Colorado State University researchers conduct a seepage measurement test to check how much water is seeping from the Catlin Canal in eastern Colorado.
Ahmad Abdur Rehman Lund
“We’re still exploring how effective [the xanthan gum] is, what factors influence that efficacy, and the best methods for applying it,” says Gates. But he’s optimistic about the technique’s potential for improving water quality in Colorado and beyond. “It’s a massive problem involving millions of miles of earthen canals worldwide,” says Gates. Polymer canal linings represent an economically viable upgrade, Gates concludes.
Managing for Quality
Lining all of Colorado’s earthen canals may be a long-shot remedy for water that’s impaired by irrigation, but it’s not the only strategy capable of improving the purity of our streams and aquifers. An array of best management practices (BMPs) are proving to make measurable gains in water quality—but farmers find some more enticing than others.
“If I were giving away sprinklers, I’d have a line down the street with farmers that want to sign up,” says Jack Goble, general manager of the Lower Arkansas Valley Water Conservancy District, which provides support to farmers along a stretch of river that is battling elevated salinity, selenium, arsenic and uranium levels. But a typical 120-acre center-pivot sprinkler costs upwards of $120,000 thanks, in part, to continuing supply chain interruptions and price hikes. Sprinklers require little labor but deliver impressive efficiency—90%, compared to 40% efficiency for flood irrigation—meaning more water is used by crops and less water is lost to evaporation or returns to the river or groundwater aquifer. And sophisticated technology, such as GPS-guided water application, accurately meets crops’ needs—even when soil variations within the same field mean that some plants should receive one inch of irrigation while others require 1.5 inches. That precision benefits such crops as sweet corn, which topples easily when its roots sit in soil that’s loosened by too much water. Limiting the amount of applied water that ends up filtering back to streams and groundwater also reduces the potential for migrating pollutants.
Sprinklers’ expense prevents them from becoming universally adopted, but so does their broadcast pattern. Generally, sprinklers are designed to distribute water over neat geometric shapes like squares and circles. Irregular or odd-shaped fields, commonly found in Colorado’s mountain communities, are harder to serve with sprinklers. And some crops don’t thrive beneath the showers. “Broccoli doesn’t like heat, so it does well in the cooler microclimate of the sprinkler,” says Robert Sakata, a farmer near Brighton who also serves on the board of directors for various agencies and organizations including the Colorado Water Conservation Board, the Colorado Fruit and Vegetable Growers Association, and the National Onion Association. His onions, however, grew bigger when furrows delivered their water. “Why? I’m not sure,” says Sakata, “But I know the yields.”
Drip irrigation, which strings seep hoses through the soil at the level of plants’ roots, is even more expensive—and efficient. It’s what Sakata would install if money were no object, because even sprinklers can’t equal drip’s ability to match plants’ water needs. But crop rotation, where crops are altered from season to season to avoid depleting soils’ nutrients, complicates hose layouts, which must be adjusted to each plant’s preferred depth. Drip also assumes continuous water delivery, which Sakata can’t count on. During drought periods, when ditch shares are limited, ditches deliver water to growers by section, so that one producer might get water for two days, then no water for the following four.
Still more BMPs include installing grass filter strips, like the ones at Lempka Farms. Lining the small ponds that growers use to feed their sprinklers also reduces the flow of contaminants, says Goble, whose district just lined its eighth sprinkler pond.
Projects that reduce the amount of tilled soil in each field also improve water quality by trapping more of the applied water in the soil and limiting contaminated runoff. Since 2010, when Colorado farmers asked CSU to create a demonstration plot measuring the benefits of conservation tillage–which includes no-till, low-till and other tillage practices that reduce erosion by protecting the soil surface–various metrics have borne out its benefits for efficiency, water quality and soil health. Farmers are readily adopting the new tillage strategies because they have fewer costly inputs: Less water requires less fuel and labor, and improves soil health, says Wardle. “They see that if they do this well, they’ll make more money.”
Some producers also adopt BMPs to avoid mandatory oversight. In 2012, Colorado passed Regulation 85 to address dispersed, “nonpoint” sources of nutrient pollution related to agricultural operations. Point sources, such as wastewater treatment plants and animal feed lots, were already regulated. State regulators urged growers to voluntarily adopt practices that improve water quality, and this year, they’ll review whether sufficient gains have been made to forestall additional rules.
“We have made good progress,” says Nicole Rowan, director of the Water Quality Control Division of the Colorado Department of Public Health and Environment. She says the education campaign that the state conducted with entities such as CSU and the Colorado Department of Agriculture has motivated many producers to pursue federal cost-share opportunities that help clean up water sources. Consequently, Rowan does not expect to recommend that the state adopt compulsory participation or additional regulation. Besides, she notes, other states across the nation, including those along the Mississippi River, are also inviting voluntary compliance from agricultural producers and have achieved promising water quality improvements.
In Colorado, the Lower Gunnison isn’t the only waterway to make measurable gains in quality. The South Platte has also improved its scorecard, says Rowan. In 1989, when CSU began its agricultural water quality program database with sampling conducted along that river in Weld County, 83% of sampled wells exceeded acceptable standards for nitrate (a contaminant associated with fertilizer runoff), but that fell to 55% in 2020.
There is some conflict between projects that improve water quality by reducing seepage or boosting efficiency and the need to protect the flow of water to downstream users and the environment.
Significant habitat has developed around ditch seepage—irrigation-induced wetlands and other vegetation allow return flows to recharge groundwater in places and provide rich habitat to birds and wildlife. Without seepage and excess irrigation water, those environments would die off.
Then there are legal and financial barriers that can disincentivize water efficiency. For example, Goble often fields concerns from growers who are reluctant to line their sprinkler ponds, because doing so could forfeit a pond seepage credit they receive, potentially increasing their need to purchase additional replacement water to stay in compliance with a set of Irrigation Improvement Rules specific to the region. Producers in the Arkansas River Basin who become more efficient with irrigation have to pay in augmentation costs. These rules were instituted by the Colorado State Engineer in 2011 to ensure that downstream water users aren’t shorted and ensure compliance with the Arkansas River Compact. The compact with Kansas decrees that the Arkansas must maintain the same flows that it delivered in 1949. “Water seeping from sprinkler ponds goes back to the river and heads downstream to Kansas,” explains Goble.
In other watersheds, Colorado’s water laws sometimes mean that producers who improve efficiency risk losing some of their water rights and compromising the dollar value of their properties. “It’s a nuanced issue, and can be a real barrier to efficiency improvements, at least in some locations,” says Wardle.
As always with water solutions, magic wands are few. But fractions matter, and widespread implementation of broad-spectrum management practices can transform Colorado’s water quality. All it takes is a growing number of Larry Lempkas who are curious about their impact. “Some people are more worried that somebody is going to pinpoint them as a problem,” admits Lempka, who spreads a contrary message. “These studies are not policing anything,” he says. “They let us know where we can make a difference.”
A freelance writer living in Steamboat Springs, Colo., Kelly Bastone covers water, conservation and the outdoors for publications including Outside, AFAR, 5280, Backpacker, Field & Stream, and others.